Exploring Quantum Computers: Principles and Applications
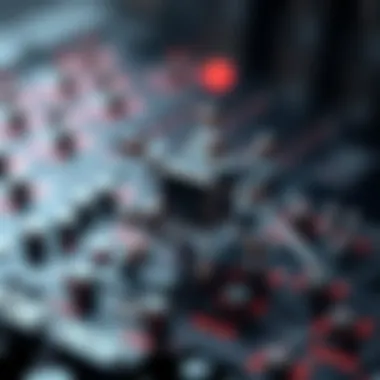
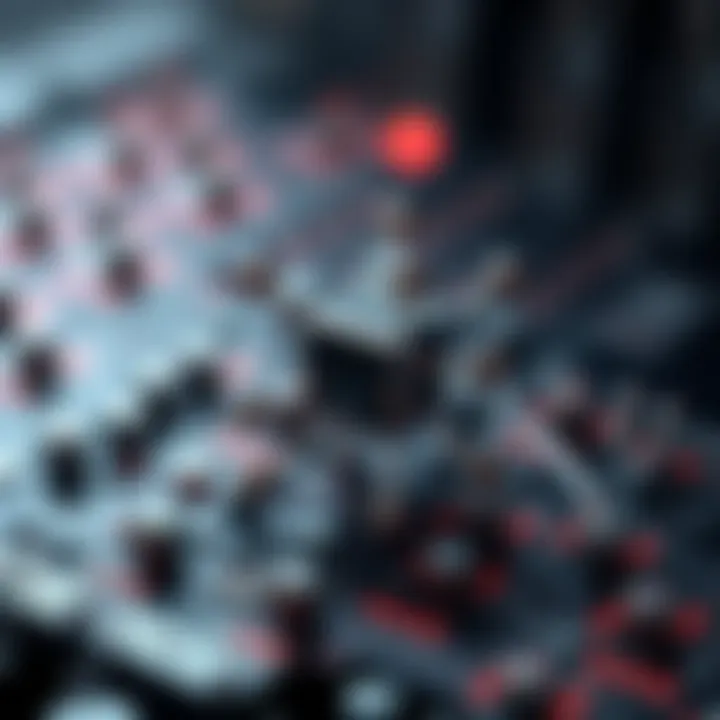
Intro
In an era where technology is evolving at breakneck speed, the emergence of quantum computing stands out as a pivotal advancement. Unlike classical computers that operate on binary bits, quantum computers utilize qubits, which can exist in multiple states simultaneously. This capability sets the stage for unparalleled processing power and efficiency. As we forge ahead, understanding these principles becomes paramount not just for tech enthusiasts but also for investors, educators, and professionals working at the frontiers of science.
This article will unravel the intricacies of quantum computing, beginning with the fundamental principles that underpin this revolutionary technology. By comparing it to classical computing, we aim to highlight what makes quantum different, while also delving into concepts like superposition and entanglement. Furthermore, we'll explore the myriad applications and potential impact quantum computing may have across various domains, including healthcare, finance, and artificial intelligence. Let's embark on this enlightening journey into the heart of quantum technology.
Foreword to Quantum Computing
Embarking on the journey into quantum computing opens a doorway to a realm where traditional notions of computation blur. It doesnāt merely represent a new chapter in technology; it signifies a potential rewrite of the very rules that govern information processing. Understanding quantum computing is essential for those wishing to grasp how future technologies might unfold and reshape industries. This area of study holds the promise of solving complex problems much faster than classical systems, addressing challenges that currently stall progress in fields ranging from cryptography to drug discovery.
At the heart of quantum computers lies a revolutionizing principle: quantum bits, or qubits. Unlike classical bits that exist solely as zeros or ones, qubits can embrace a state of superposition, representing multiple values at once. This feature alone allows quantum systems to perform calculations at extraordinary scales and speeds.
Importance of This Exploration
Delving deep into the foundations of quantum computing not only enhances our understanding of its operational mechanisms but also illuminates its implications across various sectors. Whether one is an investor eyeing the next big tech breakthrough, an enthusiast keen on innovations, or a developer wanting to grasp new programming languages derived from quantum mechanics, the knowledge gleaned from this exploration equips the audience with the insights needed to navigate this evolving landscape, maximizing opportunities.
Additionally, pondering the historical context of quantum computing reveals a narrative rich with exploration and discovery, charting a course from theoretical ideas to tangible implementations. Understanding the journey aids in appreciating the complexities and potential of quantum technology today.
Principles of Quantum Mechanics
The realm of quantum mechanics serves as the foundation for understanding quantum computing. It introduces various ideas that defy common intuition and challenge our classical perceptions of physics. At its core, quantum mechanics reveals that the microscopic world operates under rules distinct from those of macroscopic objects. This section will delve into fundamental concepts like wave-particle duality, quantum superposition, entanglement, and the measurement problem. Understanding these principles not only shines a light on how quantum computers operate but also paves the way for their transformative potential across various fields.
Basic Quantum Concepts
Wave-Particle Duality
Wave-particle duality is a cornerstone concept in quantum mechanics, illustrating that every particle or quantum entity can exhibit both wave-like and particle-like properties. This duality is not merely an academic curiosity; it has profound implications for our understanding of reality. For instance, light can behave as a wave, displaying interference patterns, while in other contexts, it acts like a particle, e.g., photons interacting with surfaces.
The characteristic of this duality reflects the unpredictable nature of quantum entities. By accepting that something can be two things at once, we widen our scientific horizons. While this might be confusing, it's crucial for grasping quantum computing. The benefit here is undeniable: it provides a richer paradigm for processing information. In quantum computing, this dual nature means that data isn't just binary; it can be in multiple states simultaneously.
However, embracing wave-particle duality does come with its challenges. For many, it raises more questions than answers. The very essence of reality seems to become blurred, making it a tough pill to swallow for some scientists and enthusiasts alike.
Quantum Superposition
Moving on to quantum superposition, this principle states that a quantum system can exist in multiple states at once until it's measured. This characteristic is pivotal to the functionality of quantum computers. Unlike classical bits, which can only be in the state of 0 or 1, quantum bits, or qubits, can exist simultaneously in both states. Essentially, this allows quantum computers to perform various calculations at once, vastly improving computational power.
The main attraction of superposition lies in its potential for efficiency. This trait can lead to significant optimization in processes such as cryptography, optimization problems, and simulations of complex systems. However, itās crucial to acknowledge the delicate nature of superposition as well. Systems in superposition can be easily disturbed by their environment, leading to decoherence, where they lose their quantum behavior and revert to classical states.
Quantum Entanglement
Quantum entanglement is another remarkable feature of quantum mechanics, affecting particles that become intertwined such that the state of one immediately influences the state of another, regardless of distance. This phenomenon is often referred to as āspooky action at a distanceā by Albert Einstein, indicating that the behavior of entangled particles is fundamentally non-local. Such relationships can lead to improvements in quantum communication and encryption.
Entanglement allows for faster than light coordination between particles, enabling mechanisms like quantum teleportation, hence the potential for revolutionary advances in data transfer and security systems. However, the strange nature of entanglement poses inherent questions about the very fabric of spacetime and causality.
Quantum Measurement
At the heart of quantum mechanics lies the measurement problem. When we measure a quantum system, we invariably change it. The act of observing forces the system to choose a specific state from its possible superpositions, collapsing the wave function. This peculiar interaction raises significant philosophical debates about reality and observation.
The consequences of quantum measurement extend directly to quantum computing. In a computation context, measurements are unavoidable yet can disrupt the computational process. Careful consideration must be taken during the measurement phase to avoid loss of information.
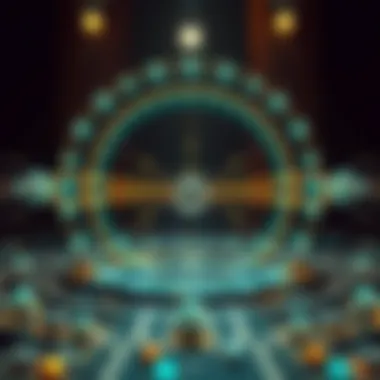
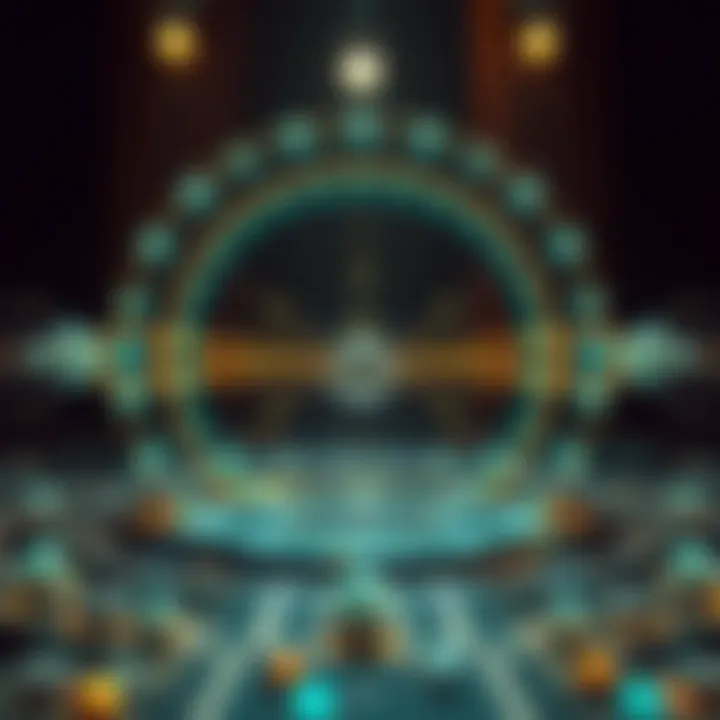
In summary, the principles of quantum mechanics paint a fascinating, if complex, picture of the natural world. By mastering these concepts, one can better comprehend how quantum computers operate and appreciate their potential residing beyond the horizon.
Architecture of Quantum Computers
The architecture of quantum computers is pivotal for understanding how these advanced systems operate and how they differ from traditional computing systems. At the heart of quantum computing lies the qubit, which acts as the fundamental unit of information. Unlike classical bits, which can exist in a clearly defined state of either 0 or 1, qubits have the unique ability to exist in multiple states simultaneously, thanks to the principles of quantum mechanics. This feature allows quantum computers to process information in fundamentally different and more efficient ways, enabling calculations that classical computers may find impractical or impossible.
Qubits: The Building Blocks
Types of Qubits
There are several types of qubits, each with its own unique characteristics. Among the most widely discussed are superconducting qubits, trapped ion qubits, and topological qubits. Superconducting qubits are advantageous because they can be created and manipulated at relatively low temperatures, making them a popular choice for many leading quantum computing projects. On the other hand, trapped ion qubits offer high coherence times, meaning they can preserve their quantum information for longer durations, which is crucial for error correction and complex calculations.
The key characteristic of these types is their ability to maintain coherence while allowing flexibility for manipulation, making them favorable in both experimental and commercial quantum computing environments.
However, each type of qubit comes with its own advantages and disadvantages. For instance, while trapped ions are very stable, they require sophisticated laser setups, which can complicate their operational implementation.
Physical Implementations
The physical implementation of qubits relates directly to how these quantum systems are built and operated. There are many avenues to realization, each contributing to different technical possibilities or limitations. For example, superconducting qubits utilize circuits formed from superconducting materials, allowing current to flow freely without loss, making it easier to create and control qubit states. Alternatively, silicon-based qubits can potentially be integrated with existing classical electronics, paving the way for hybrid systems to emerge.
Choosing a physical implementation hinges on various considerations, including scalability, error rates, and integration capabilities. A unique aspect is the potential for quantum supremacy, which is the moment when quantum computers can perform calculations beyond what classically feasible systems can achieve. This goal leads researchers to constantly explore and debate the most effective implementation strategy. However, these innovations come with challenges, such as environmental noise and temperature sensitivity, which can impact coherence.
Quantum Gates and Circuits
Quantum gates are the heart of quantum circuits, responsible for performing operations on qubits. These gates manipulate the states of qubits to perform complex calculations. Unlike classical logic gates, quantum gates are reversible and operate under the principles of superposition and entanglement. The arrangement of these quantum gates within quantum circuits determines the overall functionality of the quantum computing system. By constructing various circuits, quantum computers can solve highly complex problems that are beyond the reach of classical computers.
Error Correction in Quantum Systems
Error correction is one of the most significant challenges faced in quantum computing. Due to the fragile nature of qubits, they are prone to errors caused by environmental factors like temperature fluctuations and electromagnetic waves. Quantum error correction codes are vital to ensure the reliability of quantum computations. These codes help to detect and correct errors without directly measuring the qubits, preserving their superposition state. As the field progresses, developing efficient error correction methods will ultimately dictate the success of scalable quantum computing systems.
Comparing Classical and Quantum Computing
In the expanding realm of technology, the comparison between classical and quantum computing is crucial. Understanding these differences allows us to grasp how quantum computers can potentially revolutionize industries by enhancing processing capability and solving complex problems that are currently unfeasible with classical systems. As we venture into this intricate subject, itās essential to appreciate not just the technical specifications but also the implications these differences hold for the future.
Key Differences
Data Processing
When discussing data processing, a primary aspect lies in how these two systems handle information. Classical computers operate on binary digits (bits), which can exist only in a state of 0 or 1. In contrast, quantum computers utilize qubits. This fundamental difference means that a qubit can represent both 0 and 1 simultaneously through the phenomenon of superposition. This concurrency allows quantum computers to process vast amounts of data much faster than classical depictions.
One key characteristic of data processing in quantum computing is parallelism. Classical computers handle tasks linearly, one after the other, which can be time-consuming. However, through superposition, a quantum computer can execute various computations at once, making it an exhilarating choice gradually reshaping data-heavy domains like pharmaceuticals, logistics, and finance.
However, with this potential comes challenges. Although the theoretical models are promising, building a robust quantum processor that can maintain qubit stability and avoid decoherence is a gargantuan task. Without overcoming these hurdles, the advantages in data processing remain largely untapped.
Speed and Efficiency
Speed and efficiency are twin pillars that are often scrutinized in the context of performance comparison. Classical computers, while powerful, eventually hit a wall when it comes to calculating intricate algorithms required for tasks such as cryptography or large-scale simulations. These tasks can take an eternity to completeāthink of them as attending a very slow dinner party where each course takes forever to arrive.
In contrast, quantum computing promises exponential speed-ups in computations. For instance, Shor's algorithm, which can factor large numbers in polynomial time, showcases the potential of quantum computers to solve problems previously deemed impractical for classical architectures. This speed advantage could disrupt fields like security, where encryption relies heavily on the factorization difficulty. Still, itās vital to tap into these efficiencies judiciously as real-world implementations face significant resource consumption during their calculations due to error rates and the need for error correction mechanisms.
Limitations of Classical Computers
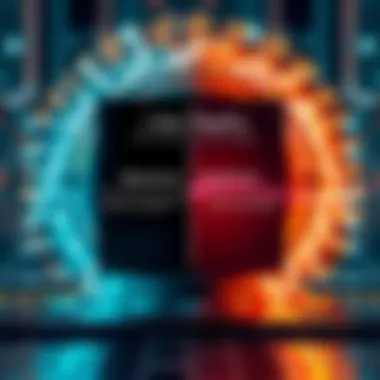
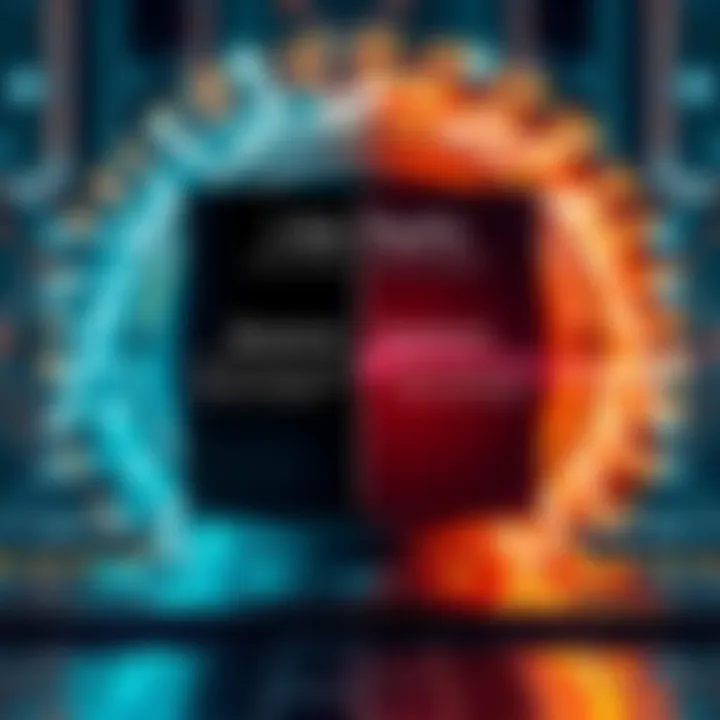
Despite their solid place in our current technology landscape, classical computers do have limitations that often impede innovation. For instance, they struggle with two primary issues: scalability and speed constraints. As problems grow in complexity, the linear nature of classical computation leads to a bottleneck, often requiring disproportionate levels of power and time to yield solutions. This means, in practice, that many potentially beneficial advancements remain just over the horizon, requiring either groundbreaking new algorithms or entirely novel architectures.
In summary, a lucid understanding of how quantum computing contrasts with classical systems leads us into unexplored territories of innovation. As we step further into this discussion, itās clear that the future promises not just upgraded speed and efficiency, but also a rethinking of how we approach computations and problem-solving across diverse spectrums.
Applications of Quantum Computing
The advent of quantum computing has unleashed a wave of possibilities that could reshape various industries. This section digs into the practical applications of quantum computing, shedding light on some prominent areas where the technology is making notable strides. It highlights the transformative potential of quantum systems and how they tackle problems that were once thought to be unsolvable with classical computers.
Cryptography
Quantum Key Distribution
Quantum Key Distribution (QKD) plays a critical role in the security domain, offering a framework for secure communication that classical systems cannot match. At its core, QKD utilizes the principles of quantum mechanics to enable two parties to generate a shared, secret key for encrypted communication. One of the most significant characteristics of QKD is its reliance on the laws of quantum physics, which allow it to detect any eavesdropping attempts. This unique feature makes it increasingly valuable as cyber threats become more sophisticated.
Moreover, QKDās ability to ensure confidentiality is a game-changer. In a world where data breaches are alarmingly common, its implementation provides organizations with a strong defense against potential attacks. However, the technology isn't without its challenges. The need for specialized hardware and limitations in distance and transmission rates can pose barriers to widespread adoption. Still, as research continues and infrastructure evolves, QKD is on the path to becoming a standard in secure communications.
Impact on Current Cryptographic Systems
Quantum computingās impact on existing cryptographic frameworks is profound and significant. Current systems, which often rely on complex mathematical problems to secure data, could become vulnerable with the power of quantum machines. For instance, algorithms like RSA and ECC, upon which much of today's security rests, could be cracked efficiently by quantum computers.
This impending challenge to cryptography highlights the vital need for post-quantum cryptographic algorithms, which are designed to withstand quantum attacks. Developing these alternatives ensures that sensitive data remains protected even as quantum technology advances. One of the key characteristics here is the urgency that accompanies this transition; organizations must adapt to the threat posed by quantum computing, lest they remain unprotected. Though this daunting challenge looms, it is also an opportunity for innovation, as cryptographers now focus on creating resilient systems to safeguard our digital lives in the coming quantum era.
Optimization Problems
The realm of optimization problems is another area where quantum computers shine. These complex puzzles often arise in logistics, finance, and production environments where maximizing efficiency is paramount. Quantum algorithms, such as the Quantum Approximate Optimization Algorithm (QAOA), aim to tackle these problems where classical computers struggle, especially as complexity increases.
Through their unique structure of qubits and quantum gates, quantum computers can process numerous possibilities simultaneously. This allows them to identify optimal solutions for vast datasets much quicker than classical counterparts can. Organizations keen on interpreting intricate data can harness quantum optimization to streamline operations, minimize costs, and enhance decision-making processes.
Simulating Quantum Systems
Simulating quantum systems is perhaps one of the most visible applications of quantum computing. Traditional computers wrestle with simulating quantum phenomena due to the tremendous computational resources required. However, quantum computers are inherently suited for this task. By working with qubits that reflect quantum states, they can model complex systems with unparalleled accuracy.
This capability holds vital implications for fields such as materials science and drug discovery. For instance, researchers can create precise simulations to experiment with molecular interactions, potentially leading to breakthroughs in new materials or pharmaceuticals. Companies invested in research and development are finding that by employing quantum simulations, they can achieve insights that previously took years of experimentation, thus accelerating innovation.
In summary, quantum computing's applications stretch across various sectors, each with its unique benefits and challenges. From enhancing security through quantum key distribution to revolutionizing optimization and simulating natural processes, the technology is poised to leave a significant mark on many aspects of modern life. As developments continue, keeping a keen eye on these applications may yield insights into the future landscape of quantum technology.
Current State of Quantum Computing
Quantum computing is entering a significant phase of development, where both academia and industry are investing heavily. Understanding the current state of quantum computing is crucial for investors and tech enthusiasts, as it sheds light on the advancements being made and future possibilities. There are numerous elements to consider when examining todayās quantum landscape: research institutions spearheading breakthroughs, the involvement of tech giants, and the collaborative efforts between sectors.
Leading Research Institutions
In the sphere of quantum research, several institutions stand out as beacons of innovation. First and foremost, the Massachusetts Institute of Technology (MIT) is leading the charge. MITās research is often at the forefront of quantum algorithms and actual quantum hardware. Similarly, Stanford University has cultivated significant advancements in techniques for quantum communication and cryptography.
The University of California, Berkeley, is making strides, particularly in quantum optics, moving towards practical applications that could change the game. Meanwhile, the University of Oxford in the UK has been a vital player in qubit technology, working tirelessly to push the boundaries of what's possible with quantum bits.
Other institutions like IBM's Quantum Experience and Googleās Quantum AI both exemplify the power of large corporations collaborating with academia. These partnerships bridge the gap between theoretical physics and practical technology, fostering innovation in quantum computing.
"Collaborative research between universities and industry is whatās pushing quantum technology from theory to reality."
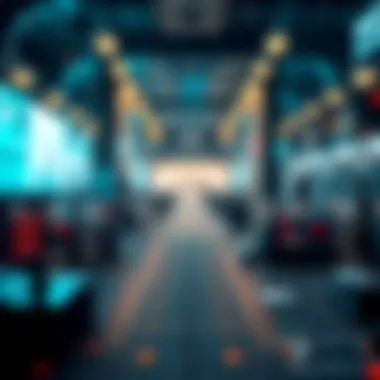
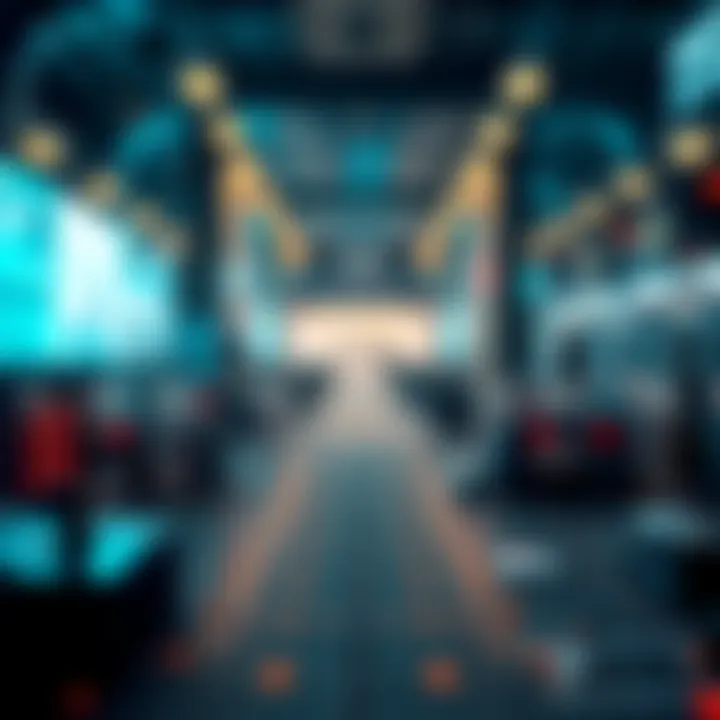
Major Players in the Industry
While research institutions lay the groundwork, industry players are translating these findings into real-world applications. Companies like IBM are pioneering efforts, with their Quantum Q System One being a key player in making quantum computing accessible for businesses.
Then there's Google, which made headlines with their Sycamore processor, achieving quantum supremacy in 2019. This marked a significant milestone in demonstrating that quantum computers can perform specific tasks much faster than classical computers.
Microsoft with its Azure Quantum platform and startups like Rigetti Computing and IonQ are also in the mix, working to democratize access to quantum resources. The growing interest among venture capitalists in quantum startups indicates a strong belief in the market potential for these technologies.
In addition, large tech firms like Amazon are providing cloud-based quantum computing solutions, bridging the gap between powerful computation and usability. This democratization of quantum technologies opens the door for smaller companies and innovators to harness quantum power, moving from research labs to practical applications.
The current state of quantum computing is marked by an exciting convergence of academic insight and industrial application, representing a dynamic ecosystem ripe for growth and exploration.
Future Perspectives
As we gaze into the crystal ball of quantum computing, it becomes clear that the future of this technology holds considerable promise and numerous uncertainties. Understanding these future perspectives is crucial, as they inform investors, educators, analysts, and developers about impending changes and potential disruptions across industries. This section aims to highlight emerging trends and potential societal impacts that could arise from advancements in quantum computing.
Emerging Trends
Emerging trends in quantum computing are like the first saplings in a vast forest, hinting at what might grow in the years to come. Companies and research institutions are investing significant resources into enhancing quantum algorithms and hardware. Noteworthy developments include:
- Advancements in Quantum Hardware: Traditional computing components have their limits, but new developments in qubit technologyālike superconducting qubits and trapped ionsāare paving the way for robust quantum systems. Companies like IBM and Google are already pushing the envelope, making headway towards a more stable and reliable architecture.
- Quantum Networking: As more quantum devices come online, the hunger for quantum communication increases. Developing quantum networks will allow these devices to communicate securely and efficiently, potentially leading to breakthroughs in fields such as data encryption and information sharing.
- Hybrid Computing Models: The merging of classical and quantum computing is anticipated. Hybrid models will harness the strengths of both worlds. Classical computers will handle straightforward tasks while leaving complex computations to quantum systems.
- Increased Accessibility: As quantum cloud services evolve, access to quantum computing will expand beyond tech giants to lesser-known startups and even academic institutions. This democratization of technology could lead to a surge in innovation.
These trends signal not merely an evolution but a revolution. As the landscape shifts, adaptability and awareness will be key for those looking to thrive in this quantum era.
Potential Societal Impacts
The ramifications of quantum computing extend far beyond the technical sphere, reaching into societal changes that touch on ethics, privacy, and accessibility. Hereās a closer look at how quantum advancements may influence society:
- Reimagining Cryptography: The impact on security measures will be profound. With quantum computers potentially able to break RSA encryption, industries relying on data security must rethink their approaches. This includes looking into quantum-resistant cryptographic methods to safeguard sensitive information.
- Transforming Industries: Fields like pharmaceuticals, materials science, and finance stand to benefit enormously from the capabilities of quantum simulations and optimization. Faster drug discovery, improved financial modeling, and efficient supply chain logistics could reshape these industries, driving global economic growth.
- Ethical Considerations: With power comes responsibility. As quantum technology progresses, ethical questions about its usage will emerge. Potential misuseālike deepfake technology powered by quantum computationāraises alarms about privacy, consent, and security. Societal debates on accountability in this new digital age are inevitable.
- Educational Reforms: An increase in quantum computing literacy will be essential. As industries adapt, educational institutions may need to revamp curricula and train a new generation of tech-savvy thinkers. This aspect brings the challenge of equitable access to quality education in advanced technologies, ensuring that all communities can benefit.
Overall, the upheaval that quantum computing may bring demands foresight and careful planning. Anticipating these changes can bolster readiness, empower individuals, and ignite innovation. As we navigate this complex landscape, staying informed and adaptive will serve society well in harnessing the full potential of quantum technology.
Closure
In this article, we explored the intricate world of quantum computing, peeling back the layers to understand its principles and functionality. The significance of concluding on this topic lies primarily in the understanding of how quantum technology is not just a fleeting trend, but a major pivot in the trajectory of computational science. As the digital landscape evolves, the necessity for speed, efficiency, and solutions to complex problems becomes paramount. Quantum computers not only offer solutions to existing challenges but also pave the way for innovations that could redefine entire industries.
Summarizing Key Takeaways
To encapsulate the crux of this article:
- Quantum computing operates on fundamental principles such as superposition and entanglement, which starkly contrast with classical computingās binary approach.
- The versatility of qubits and the ramifications of quantum gates illustrate the potential for increased computational power and efficiency.
- Real-world applications of quantum technology, from cryptography to optimization problems, signify its far-reaching implications.
- Current advancements indicate a growing interest among leading research institutions and industry players poised to harness the power of quantum computing.
Understanding quantum computing is crucial not only for those immersed in technology but also for investors and educators seeking to grasp the future of tech-driven solutions.
The Road Ahead for Quantum Computing
Looking ahead, the prospects for quantum computing are astonishingly promising. As more organizations invest in research and development, we can expect:
- Ongoing breakthroughs that enhance the stability and reliability of quantum systems.
- Further exploration into hybrid solutions that intertwine classical and quantum computing, optimizing resource utilization.
- Potential shifts in various sectors, including healthcare, logistics, and artificial intelligence, leveraging quantum algorithms for better predictive models.
The road will not be without bumps; challenges remain, particularly in error correction and creating robust algorithms. However, the future of quantum computing, filled with emerging trends and profound societal impacts, holds a captivating allure for those willing to embark on this transformative journey.
The fusion of quantum mechanics with practical computing has the potential to change our reality, speeding through complex calculations in ways classical computers could only dream of.
As we wrap this discussion, one canāt help but feel that we are on the brink of a new era, with quantum computers potentially redefining what we understand as computation itself.
For more information, you may explore resources such as Wikipedia on Quantum Computing or delve into quantum-related discussions on Reddit.